What is flooded rice?
Flooded rice systems, a type of paddy rice, are irrigated, rainfed, or deep-water rice production systems with a growing cycle of approximately 105-150 days, depending on the variety. Flooded rice is cultivated for up to two or three seasons each year. Flooding rice fields improves the growth of most varieties, reduces vulnerability to uneven rainfall, and produces higher yields relative to dry soils by reducing pests and weeds. Over 90% of the world’s rice is produced in Asia (Fig. 1) by countries such as China, India, Indonesia, Bangladesh, Vietnam, Thailand, Myanmar, and Japan, and the majority of this production is flooded rice.
The water regimes in flooded rice fields are diverse, but can be narrowed to four types: (i) permanent flooding, (ii) shortened flooding, (iii) intermittent flooding, and (iv) periodic moistening. Water regimes are determined by the environment (through climate and natural flooding) and by humans (through water management). Environmental or natural flooding is determined by the biophysical environment (e.g., extreme rainfall during the monsoon season). Human-induced flooding is either determined by farmers who choose how much and how long to flood, or by existing collective irrigation structures that determine flooding periods (e.g., the government opens one levy and all farmers’ fields are flooded with no individual control). These influences sometimes vary even by season.
Flooded rice systems can include, and be affected by, nutrient management, water management, agroforestry, open burning, livestock, and energy use for irrigation or processing. Land-use change may occur to expand flooded rice areas. Food loss and waste (FLW) and rice straw recycling are other elements relevant to GHG emissions management.
Contributions to emissions
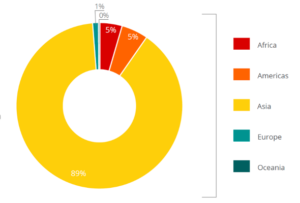
Flooded rice systems emit significant amounts of methane (CH4). Although estimates vary and have high uncertainty, flooded rice produces approximately 493-723 Mt CO2e yr-1, about 9-11% of anthropogenic emissions in the agriculture sector globally. Although, independent analyses suggest that emissions from flooded rice may be at the lower end of the estimated range. Emissions in non-Annex I countries represented more than 95% of the total, in direct relation to shares of cultivated paddy rice area (Fig. 1). Emissions are sustained only under flooded conditions.
Emission intensities, or emissions on a per-product basis (e.g., Mg CO2e per kg of grain), for flooded rice varies by region. Emission intensities for flooded rice are lowest in Oceania and Asia (0.78 and 0.89 kg CO2e kg product-1, respectively) and highest in Europe (3.2 kg CO2e kg product-1).
Mechanisms
Greenhouse gas (GHG) emissions from flooded rice cultivation predominantly consist of methane (CH4) produced from the anaerobic decomposition of organic matter in rice fields, which escapes into the atmosphere by diffusive transport through the rice plants during the growing season. As flooded rice fields are often inundated or saturated, microbially-mediated decomposition rates are comparatively slower than upland environments. This lack of oxygen, which is the most preferred electron acceptor for microorganisms, causes facultative anaerobic bacteria and anaerobic bacteria to utilize alternative electron acceptors, resulting in different products emitted. In the case of CH4 production, anaerobic and facultative microbes utilize carbon dioxide (CO2) as the alternative electron acceptor, known as methanogenesis. The rate at which these alternate electron acceptors are consumed depends on their relative abundance, the quantity and quality of organic compounds (electron donor), and the microbial populations present.
Allowing flooded rice fields to drain permits soil aeration and oxygen to enter the soil profile, reducing the quantity of CH4 produced during the growing season. See Mitigation Strategy and Options below.
Nitrous oxide, a potent GHG, can also be emitted from flooded rice systems via denitrification when fields are fertilized with nitrogen (see Nutrient Management).
Factors affecting emissions
The amount of CH4 produced from flooded rice systems is predominantly regulated by the substrates available to methanogens (forms of methane) and the influence of environmental factors.
- Organic amendments (e.g., fresh biomass, rice straw from the previous harvest, compost, or manure) have different effects on the amount of CH4 emitted, depending on the type and amount applied.
- Environmental factors regulating CH4 production include soil texture, climate and weather, and agricultural management practices (e.g. water regime, fertilization, tillage regime, and rice variety/cultivar).
Water regime, as it affects soil redox potential, is the most important management decision since the electron activity of the soil decreases after flooding. The redox potential of a soil must be below -150 mV for CH4 production.
Mitigation strategy and options
1. Adopt water drainage techniques:
- Reduce the flooding period in paddy rice fields to interrupt the anaerobic decomposition of inundated soil and organic residual material that releases CH4. Drainage of flooded rice fields has also been shown to inhibit methanogens while stimulating methanotrophs (microbes that metabolize CH4 as their energy source).
- Adopt improved water management techniques. For example, the alternate wetting and drying (AWD) technique typically results in a 30-70% reduction in CH4 emissions by inhibiting methanogenesis while conserving 30% of irrigation water without compromising yields. “Safe” AWD requires draining only to ~10-15 cm below the soil surface to ensure that the plants have stable access to water.
- Midseason drainage (MSD) involves the removal of all surface water from the rice crop at mid to late tillering for 10-14 days, allowing the soil to dry, crack, and re-aerate.
2. Adopt improved management practices:
- Dry seeded rice leaves soil aerobic most of the season without compromising yields.
- Laser land leveling reduces GHG emissions by conserving energy, reducing cultivation time, and improving input-use efficiency with the potential to enhance rice yields by improving field conditions. This practice should be performed prior to the AWD technique.
- Follow the “One Must Do, Five Reductions” technology package. “One Must” relates to using certified seeds and “Five Reductions” relates to reducing (i) seed rate, (ii) nitrogen application, (iii) pesticide use, (iv) water use (e.g., AWD and MSD), and (v) post-harvesting losses (see Case Study below).
- Compost rice straw residues rather than burning residues.
- Apply organic inputs to dry soil in the off-season.
- Combine the above strategies with direct seeding in zero-tillage systems; adding soil amendments such as calcium carbine, calcium silicate, phosphogypsum, and biochar with urea fertilizer; and cover crops.
See the IRRI information site for a comprehensive summary of mitigation options.
Case Study
Livelihoods and Food Security Trust Fund for Burma (NUTSEM) is a ten-year program funded by the UK Department for International Development (DfID, now Foreign, Commonwealth and Development Office (FCDO)) and is implemented in the Ayeyarwady Delta region of Burma. The program began in 2010 to improve rice production’s income and nutrition status for 1.63 million people in Burma. To date, the NUTSEM program interventions in the Delta region have supported the adoption of good agronomic practices (GAPs) in the flooded rice value chain in over 35,000 hectares (ha). NUTSEM has promoted major GAP interventions such as increasing farmers’ access to improved seed varieties that require less water and reduce the flooding period needed from 140 days to 120 also reducing the use of nitrogen fertilizer from 316 to 158 kg of urea ha-1 year-1.
The GHG emissions associated with the flooded rice value chain involved in the NUTSEM program have decreased by 46% per ha and 60% per ton of grain produced. Specifically, the reduction in the use of N-fertilizer reduced emissions by 0.67 tCO2e ha-1 year-1 while the use of improved seeds (short growing season and high-quality varieties) promoted a further emissions reduction of 0.23 tCO2e ha-1 year-1. Together, these GAPs have increased productivity by 35% (1.47 to 1.99 t ha-1 year-1). A further reduction in the flooding period to 90 days is expected in the coming years once new short-growing varieties are introduced in the Delta region, which will lead to a GHG emission reduction of 0.57 tCO2e ha-1 year-1.