Jump to: Factors affecting soil carbon | Mitigation strategy and options | Case Study
What is soil carbon?
Soil carbon (C) is present in two forms: organic and inorganic. Soil organic carbon (SOC) mainly results from the decomposition of organic materials such as plants, animals, and microbes in the soil. SOC affects nutrient and water storage in the soil, making it relevant to crop productivity and soil health. It is a measurable component of the soil organic matter (SOM) pool. Soil inorganic C consists of mineral forms from the weathering of usually calcareous parent material or reactions of soil minerals with atmospheric carbon dioxide (CO2).
Total global C in the upper 1 meter of terrestrial soils is approximately 2200 gigatons (Gt; 1 Gt = 1 petagram = 1 billion metric tons), which is almost three times the size of the atmospheric C pool (760 Gt) and four times the size of the biotic C pool (560 Gt). Of this, SOC is about 1550 Gt, and inorganic C is about 950 Gt (Lal 2004).
Contributions to Emissions
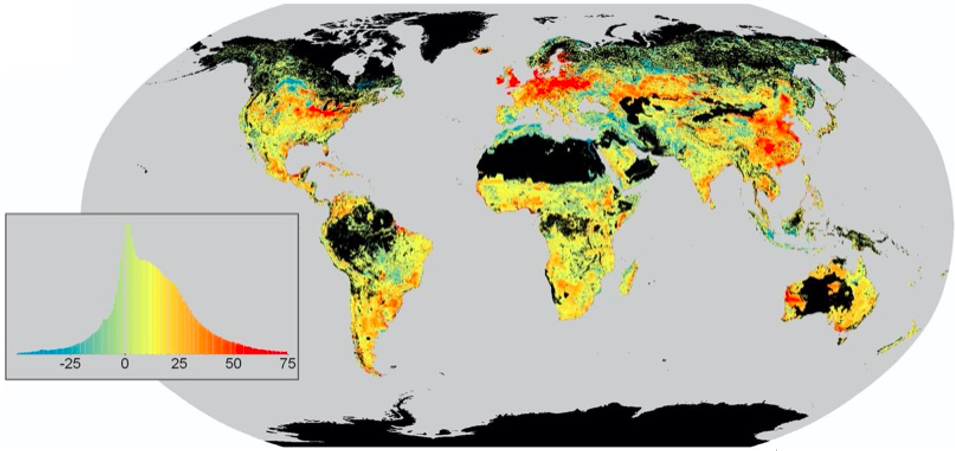
Conversion of native ecosystems to agricultural systems has depleted the global SOC pool by approximately 60% in temperate regions and 75% in the tropics (Fig. 1) or by 133 GtC for the top 2 m of soil, with most of the loss occurring in the last 200 years. Significant SOC losses have also been attributed to elevated SOC decomposition due to traditional cultivation practices and associated soil disturbance, such as conventional tillage and intensive cropping systems. Together, land-use change for agricultural production and intensive cultivation practices are responsible for one-third of global net CO2 emissions.
Soils also have the potential to decrease atmospheric CO2 concentrations. A meta-analysis by Fuss et al. (2018) demonstrated that feasible technical mitigation potential for soil C sequestration ranges from ~2-5 GtCO2 year-1 over a range of land-use types.
Mechanisms
The level of SOC stored in the soil is the result of dynamic equilibrium among mass gains (e.g., plant litter, soil roots, necromass), soil processes (e.g., microbial activity, leaching to lower soil depths), and losses (e.g., soil respiration, erosion).
Autotrophic organisms, mainly plants and photo- and chemo-autotrophic microbes, use atmospheric CO2 to synthesize organic material. Dead organic material (mainly in plant residues and exudates) is incorporated into the soil by soil organisms, leading to C inputs through organic material transformation by heterotrophic microorganisms.
The decomposition of SOC is largely a biological process in which soil organisms (mainly heterotrophic microorganisms) use SOM as an energy source. As organisms break down complex carbon compounds via enzymatic reactions in the presence of oxygen, CO2 is generated and emitted as a waste product. In addition to CO2 production during decomposition, excess nutrients, such as nitrogen (N) and phosphorus, are released into the soil profile as forms that plants can use. As successive rounds of decomposition occur, the organic matter is transformed into more complex organic molecules, called humus, and enters the passive SOM pool. The passive pool is not biologically active, meaning it provides little energy for soil organisms and is protected from decomposition, potentially resulting in net soil C gains.
Factors affecting soil carbon
- Organic matter inputs and quality: The rate of SOM accumulation largely depends on the organic matter input quality. In general, litter with a low C:N ratio, such as leguminous or hairy vetch cover crops, are easier to decompose relatively to litter with a high C:N ratio, such as wood or rye straw.
- Soil disturbance and erosion
- Depth of C location in the soil and exposure to air or oxygen
- Temperature is a key factor controlling the rate of decomposition of SOM. Decomposition rates typically increase with an increase in temperature; therefore, SOC gains are more common in cooler climates.
- Both SOC gains and losses are affected by soil moisture. SOM levels commonly rise as precipitation increases; this is due to greater biomass production, and subsequent increase in plant residues, in moist regions. However, optimal microbial activity occurs at field capacity, or 60% water-filled-pore-space, resulting in increased decomposition rates. When soils become saturated and oxygen is no longer available for aerobic microbes, decomposition rates slow.
- Soil texture, in part, determines aggregate stability or the bonding of soil particles. Clay soils tend to retain more SOM content due to their ability to form macroaggregates, which physically protect SOM from microbial attack by spatial separation.
- The composition of soil organisms regulates the C cycling in soils and depends on available food sources.
- Soil management practices such as tillage, fertilization, residue management, crop rotations, and more have strong control over the above factors by altering one or more of the biological, physical, and chemical soil properties.
Mitigation strategy and options
Adopt sustainable cropping practices that promote SOC sequestration or reduce losses.
- Reduce or eliminate tillage on well-drained soils to mitigate SOC losses as a result of soil disturbance.
- Reduce soil erosion, including the use of cover crops to reduce erosion and build up SOM.
- Diversify crop rotations.
- Retain crop residues.
- Use deep-rooted crops to promote organic matter accumulation at depth.
- Amend soils with compost, manure, biochar or other organic matter inputs.
- Integrate livestock into cropping systems (see Case Study below).
Avoid conversion of native ecosystems rich in soil C, such as forests and wetlands.
- Farmers can intensify the use of resources spatially by growing crops that take fuller advantage of sunlight, nutrients, moisture, and soil biota interactions.
Improve SOC measuring, reporting, and verification (MRV)
- Infer SOC stock changes from flux measurements rather than directly taking repeated soil samples.
- Improve spectral methods for measuring SOC stocks.
- Develop transparent, accurate, consistent, and comparable methods for MRV changes in soil C stocks, notably through new technologies, and enable standardized verification protocols at low transaction costs.
Challenges to mitigation of emissions and sequestration
- Agriculture tends to be a driver of soil C loss unless measures are taken to improve soil C explicitly.
- Soils differ in their capacity to retain additional C.
- Soils have a finite capacity to store C.
- Interventions need to be maintained, and gains are often not detectable until five years later.
- Reduced or no-till practices may increase methane and nitrous oxide emissions, both powerful GHGs, by increasing soil water holding capacity. No-till practices would benefit regions with low precipitation and on well-drained soils for minimal GHG emissions.
- Gains in SOC can be reversed if sustainable soil management practices are not maintained over time.
- Measurement, reporting, and verification (MRV) of GHG mitigation in agriculture is a challenge due to the difficulty of detecting small changes, the need for data from numerous and dispersed farms, the high cost of measurement, and lack of reliable, low-cost estimation methods (i.e., the uncertainty of modeled estimates is high).
Case study
Currently, 30,000 smallholder farmers are participating in the Kenya Agriculture Carbon Project (KACP) through 1,730 farmer groups, of which the majority of participants (70%) are women. This project by Vi Agroforestry focuses on scaling up Sustainable Agricultural Land Management (SALM) practices and generates GHG reduction credits through soil and tree carbon sequestration. The project in Kenya sequestered an estimated 344,000 tCO2e between 2010 and 2016, of which 24,788 tCO2e were sold on the carbon market to the BioCarbon Fund.
According to Amos Wekesa of Vi Agroforestry, “We have done our third round of verifications and completed three monitoring periods. We have verified 344,782 t CO2e and paid the farmers. We delivered [the] BioCarbon Fund contract and now we have Swedish Companies purchasing most of our farmers’ credits.”
The Kenya Vi Agroforestry project seeks to reduce a total of 1,950,088 tCO2e by 2030. Vi Agroforestry is working with over 100,000 smallholder farmers and around 50 farmers’ organizations in Kenya, Rwanda, Tanzania, and Uganda.